Radar Resonant Enhanced Multi-Photon Ionization
Advanced Diagnostics Navigation
- Atomic and molecular species, positive and negative ions
- Laser-Induced Fluorescence (LIF) and Two-Photon Absorption LIF (TALIF)
- Radar Resonant Enhanced Multi-Photon Ionization (Radar REMPI)
- Characterization of plasma, chemical composition and dynamic behavior
- Electron velocity distribution function, gas flow velocity and temperature
- Electric field and space potential
- Nanoparticle Diagnostics
- Surface Diagnostics
Radar Resonant Enhanced Multi-Photon Ionization (Radar REMPI)
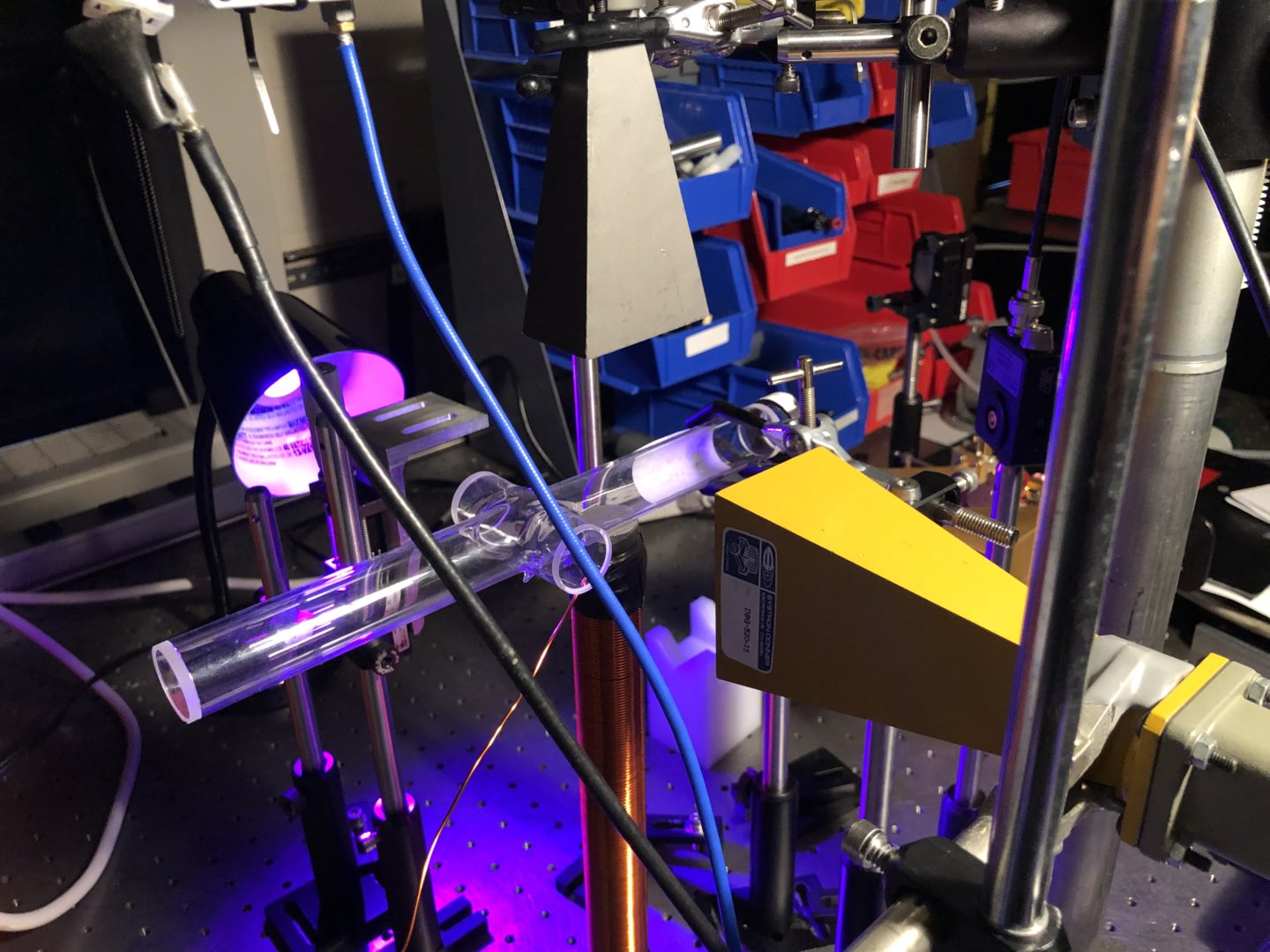
Resonance Enhanced Multi-Photon Ionization (REMPI) is a very useful spectroscopic tool for studying atomic and molecular species [1]. In REMPI, an intense laser pulse ionizes the atom/molecule and creates charges/plasma. The ionization is strongest when the photon(s) energy equals the energy difference between an excited and a ground state. Extra photons bring the energy above the ionization threshold of the atom or small molecule. In Radar REMPI, a microwave signal scatters off of the free electrons created by ionization [2]. The scattered signal is used to measure quantities such as electron number density, neutral number density, and electron loss rates. In order to monitor the plasma dynamics, it is not necessary for the laser to be resonant with an atomic or molecular transition, the Rayleigh microwave scattering can provide the temporal dynamics of plasma [3]. For example, in order to monitor the density of the negative ions, a non-resonant laser detachment process creates an increase of the electron density which can be monitored by the Radar scattered signal. By using short pulses for ionization, the temporal shape of the microwave scattering from the interrogated plasma follows the free electron population, and the peak signal can be directly related to the atomic species concentration [2]. Radar scattering on resonant and non-resonant laser induced plasma has been applied to trace species detection [4], electron loss rate measurements [5], temperature measurements in gas mixtures and flames [6, 7], as well as for time-resolved measurements of electron number density for plasma medicine applications [3] and multi-photon ionization cross-section measurements [8]. Nanosecond, picosecond, and femtosecond lasers are used to create the low density plasma detected by Radar. The microwave systems we use range from 10GHz to 100GHz, and the choice of microwave frequency is related to the size and the density of the plasma [2].
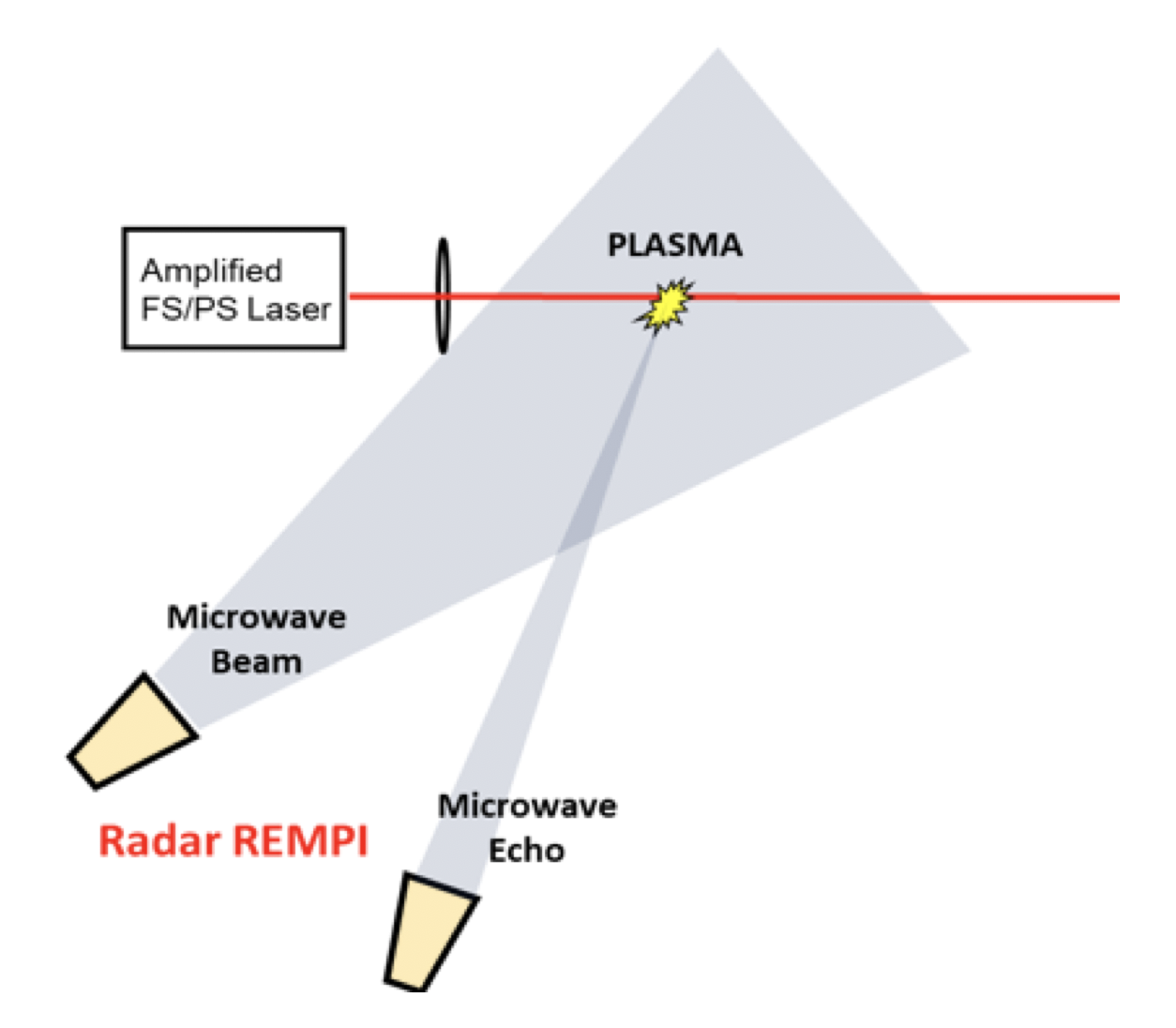
- Cool, T. A., “Quantitative measurement of NO density by resonance three-photon ionization,” Appl. Opt. 23, 1559-1572 (1984).
- Schneider, M. N., and Miles, R. B., “Microwave Diagnostics of Small Plasma Objects,” J. Appl. Phys. 98, 0033301 (2006).
- Shashurin, A., Shneider, M. N., Dogariu, A., Miles, R. B., and Keidar, M., “Temporary-resolved measurement of electron density in small atmospheric plasmas,” Applied Physics Letters, Vol. 96, 2010, p. 171502.
- Dogariu, A., and Miles, R. B., “Detecting Localized Trace Species in Air using Radar Resonance-Enhanced Multi-Photon Ionization,” Applied Optics, Vol. 50, No. 4, 2011, pp. A68–A73.
- Dogariu, A., Shneider, M. N., and Miles, R. B., “Versatile Radar Measurement of the Electron Loss Rate in Air,” Applied Physics Letters, Vol. 103, No. 22, 2013, p. 224102.
- Miles, R. B., Michael, J. B., Limbach, C. M., McGuire, S. D., Chng, T. L., Edwards, M. R., DeLuca, N. J., Shneider, M. N., and Dogariu, A., “New Diagnostic Methods for Laser Plasma- and Microwave-Enhanced Combustion,” Philosophical Transactions of the Royal Society A, Vol. 373, No. 2048, 2015, p. 0338.
- Wu, Y., Gragston, M., and Zhang, Z., “Acoustic Detection of Resonance-Enhanced Multiphoton Ionization for Spatially Resolved Temperature Measurement,” Optics Letters, Vol. 42, No. 17, 2017, pp. 3415–3418.
- Sharma, A., Slipchenko, M. N., Shneider, M. N., Wang, X., Rahman, K. A., and Shashurin, A., “Counting the electrons in a multiphoton ionization by elastic scattering of microwaves,” Scientific Report, Vol. 9, 2018, p. 2874.
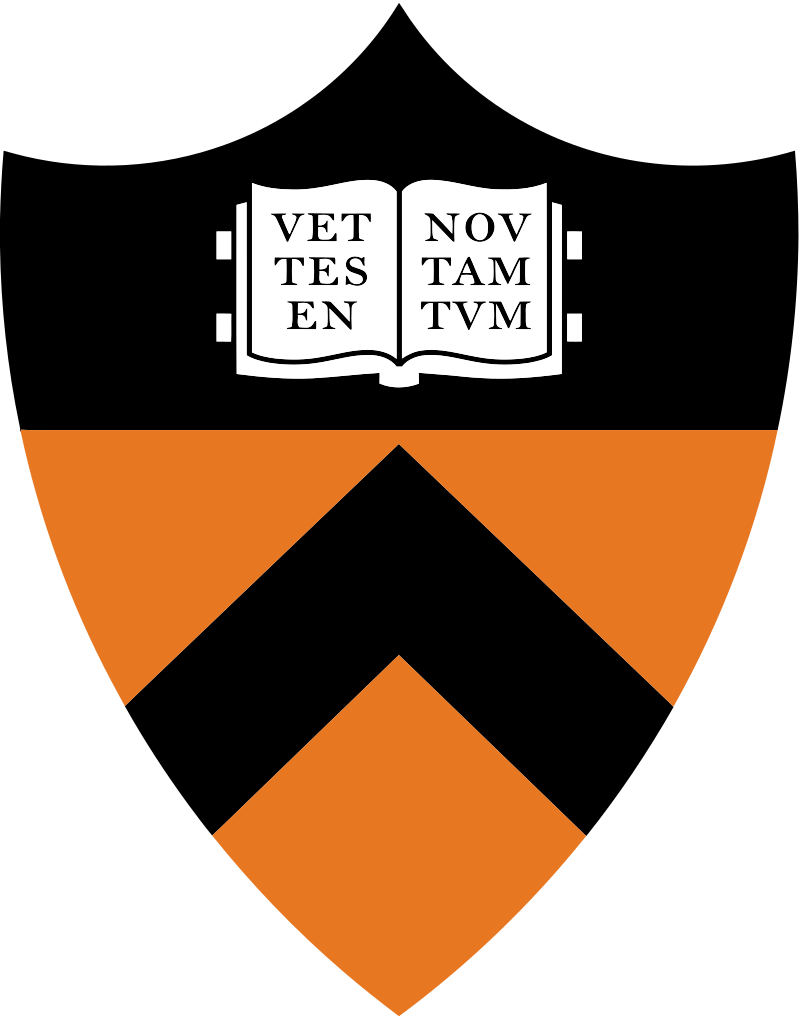