Non-equilibrium Plasmas
Plasma Sources Navigation
Non-equilibrium Plasmas
Atmospheric Pressure
FlexDBD
At PPPL, we have a flexible DBD (FlexDBD) device producing large area (1x1 cm) low temperature plasma. The PPPL FlexDBD is powered by an AC power supply, supplying HV with amplitude of 1-10 kV peak-to-peak, at frequency 20-60 kHz. The design is based on thin metal powered electrode (copper) and a thin (30 micron) meshed electrode (covered with ENIG) which is grounded. The electrodes are separated by a thin (100 micron) layer of polyimide. The openings on the mesh electrode are square-shaped, with 0.75 mm side, separated by 0.25 mm from the neighboring openings. The plasma is produced in the openings on the meshed electrode. A single device contains 100 openings, is flexible and contains cold plasma with temperature <42 C0. When operated in ambient air the plasma is chemically active as is manifested by emission of excited OH radicals.
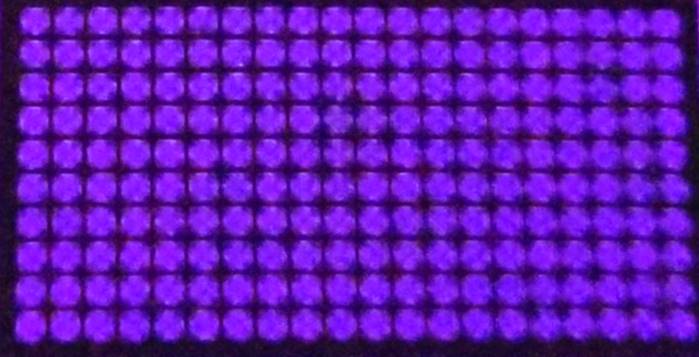
FlexDBD
Weave-DBD
The weave is made from high gauge silicon insulated flexible wires with high frequency ac high voltage applied between the wires. The discharge forms on the surface of the insulation where the interwoven wires come close enough to each other and hence, this device is a symmetrical two-barrier surface DBD, we call a weave-DBD or plasma fabric The device is flexible, can be easily produced in any size and shape such as to fit a curved surface of a lesion. The device is powered by a portable adjustable power supply. The power of the device depends on its area. For example, a 2cm2 device in disinfection experiments used <1.5 W, operating at ~40 kHz, and 25% duty cycle. The output of reactive oxygen and nitrogen species has been well characterized and contains species such as O3, OH*, H2O2, NO2, N2O, and HNO3. The relative concentration of ROS/RNS decreases with the increase in the applied voltage.
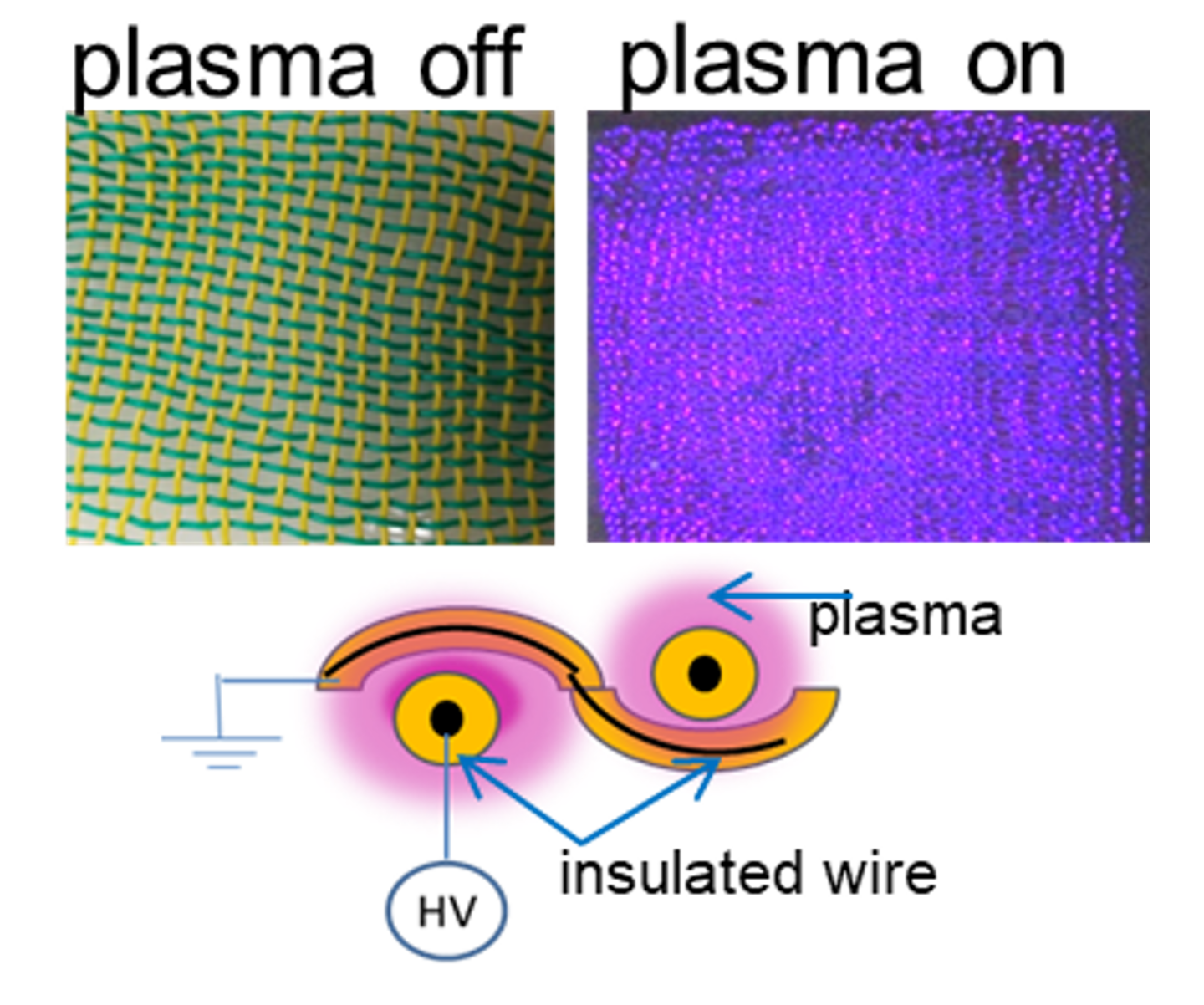
Weave-DBD
Packed-bed DBD
PCRF has packed-bed plasma reactors, a type of a dielectric barrier discharge source where at least one of the electrodes is insulated and the discharge forms in a gap between the insulator and one electrode. The discharge region is filled with insulating beads or powder. Collaborators can provide a variety of materials with different chemical compositions, porosities, and particle sizes. The packing material increases the surface area available for plasma assisted reactions and provides a matrix for a variety of catalysts for plasma assisted catalysis. The PCRF reactor also provides an optical path through the working gas allowing in situ measurements of chemical processes in the reactor.
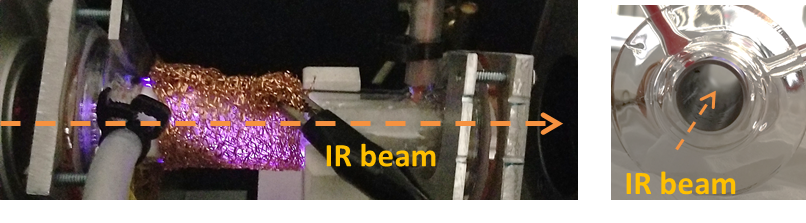
Packed-bed DBD plasma reactor
Plasma jet
At PPPL-PU facilities are equipped with a number of plasma jet devices. The plasma jet is a configuration consisting of a glass tube, with a centered powered electrode with a sharp edge, made out of tungsten. The ground electrode is a metal ring around the edge of dielectric tube. The gas flow is fed through the dielectric tube. Typical flows are on the order of 1 standard liter per minute (slm) and may contain several admixtures of different gases. The gas flows are regulated via commercial flow controllers. Another configuration of the plasma jet is when the grounded electrode is not sitting on the tube and the discharge is conducted between the sharp electrode tip and a grounded or floating surface, e.g. the liquid. In this case the glass tube can be enclosed by another tube to supply a flow of a shielding gas, which prevents air entrainment into the plasma and allows a better control of the chemistry in the plasma. PPPL-PU facilities have a number of power supplies available for sustaining plasma jets, kHz and RF.
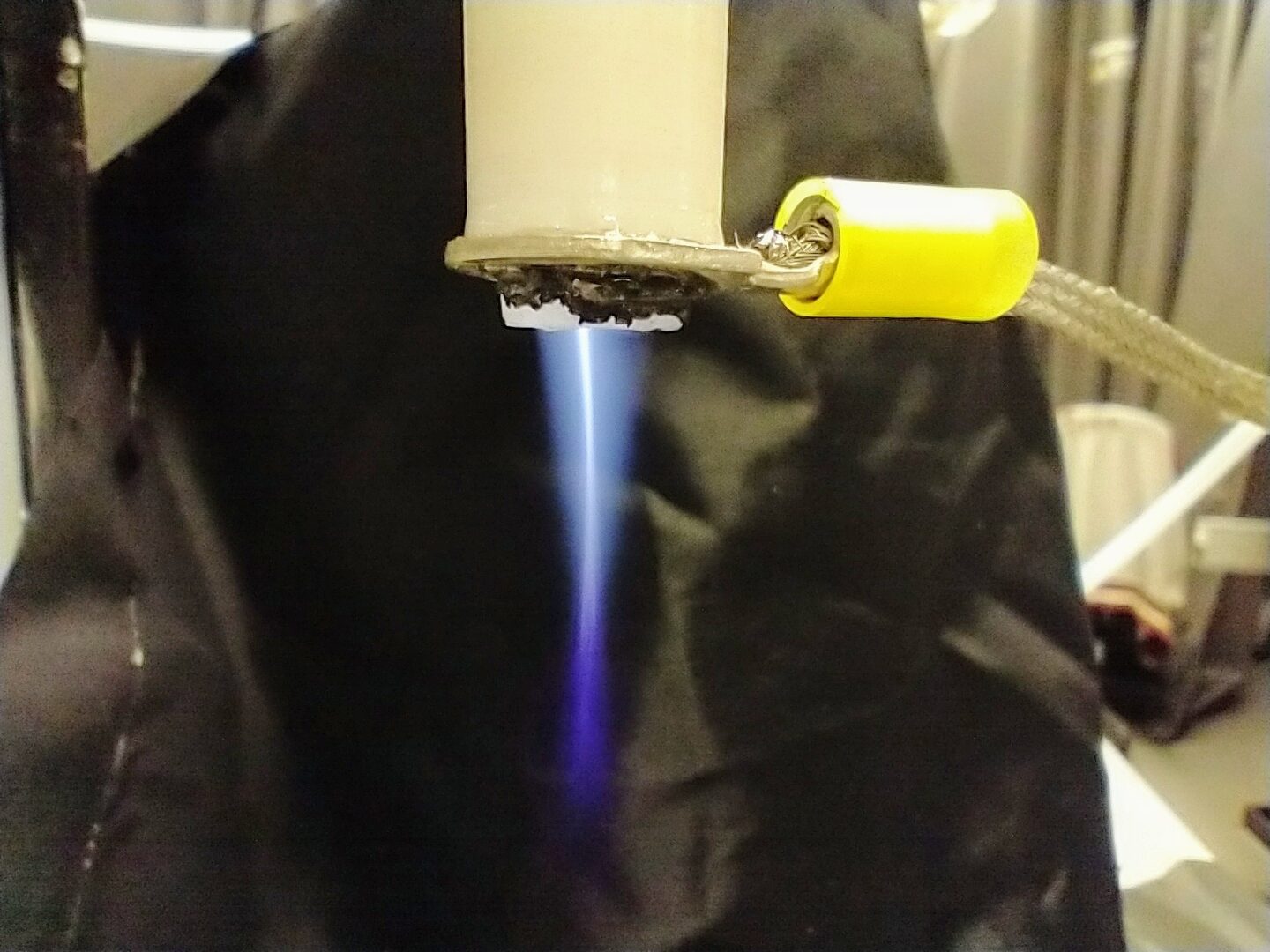
Low Pressure
Electron beam (e-beam) generated ExB plasma reactors (ExB Penning setups)
- E-beam produced plasma parameters: Te ~ 0.5-5 eV, ne ~ 109-1011 cm-3
- Reconfigurable cathodes to produce a wide range of non-thermal electrons
- Ion induced secondary electron emitting (iSEE): e-beam energy ~1-10 keV, e-beam current density, ~1-10 mA/cm2
- Thermionic cathode: ~e-beam energy 10-100 eV, e-beam current density ~100mA/cm2
- RF plasma cathode: ~10-100 eV electrons, current densities ~ 300-2000 mA/cm2
- Reactor chamber with ExB plasma accessible to wide range of diagnostics, including electrostatic probes, optical emission spectroscopy, and laser induced fluorescence
- Suitable for fundamental studies of low temperature ExB plasmas
- Suitable for research of novel material processing applications, including damageless processing of sensitive materials (e.g. 2-D nanomaterials, diamond)
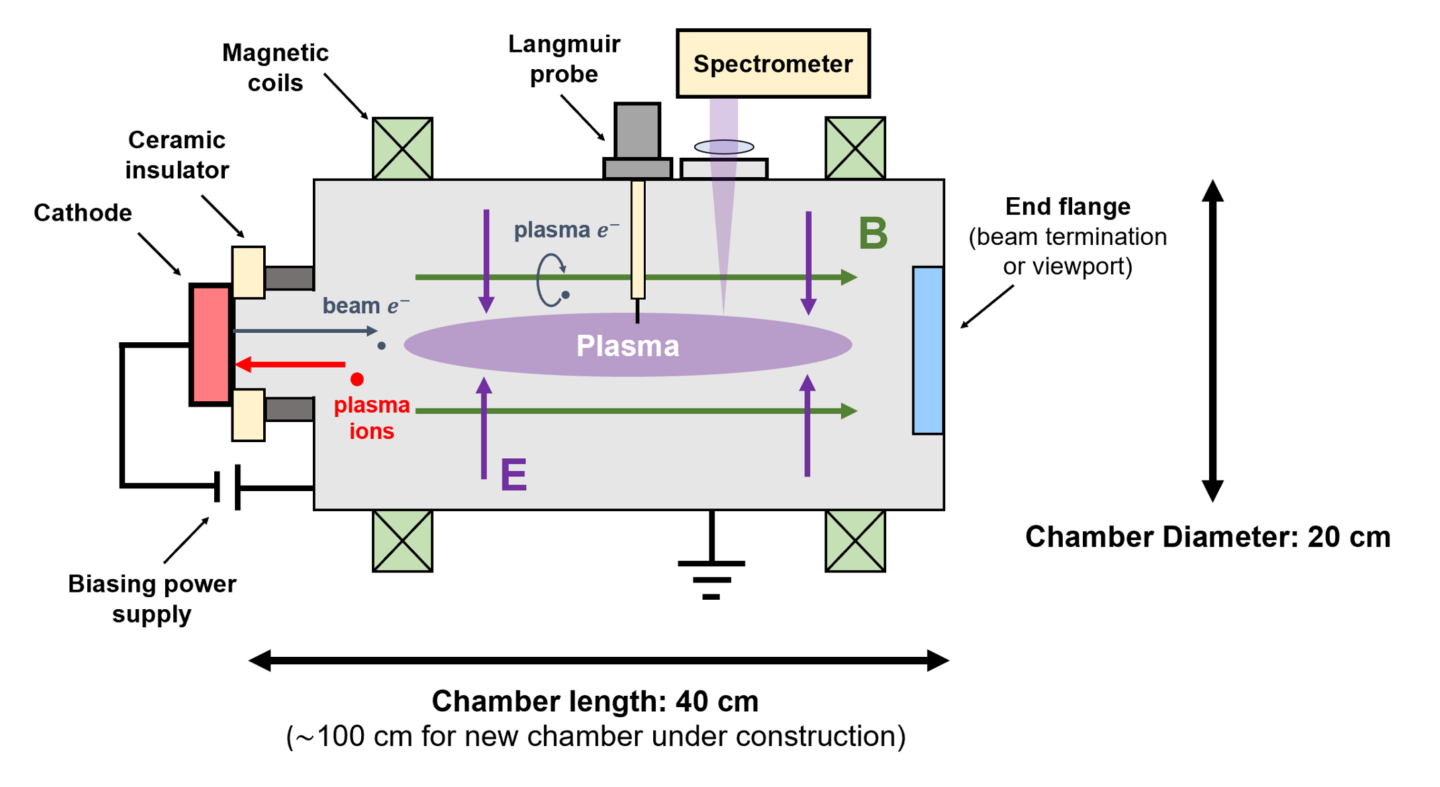
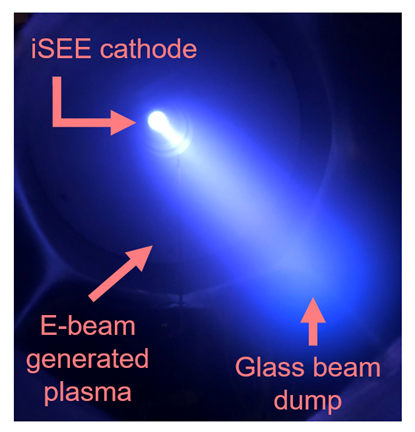
Figure 1. Diagram of e-beam generated ExB plasma chamber for low pressure radical species production (top). Image of plasma generated by iSEE electron source (bottom).
A schematic of the e-beam generated ExB plasma reactor (ExB Penning setup) is depicted in Figure 1. The reactor chamber is based on a stainless steel 10” diameter 6-way cross chamber with extensions. An e-beam is injected from a cathode along the axis of the chamber by biasing a cathode relative to the grounded chamber walls. The total length between the beam injection and the end flange (e-beam propagation length) is about 40 cm. The e-beam sustains ionization of the neutral gas in the chamber, producing a low temperature plasma. A tunable axial magnetic field, colinear with the e-beam, limits the motion of lower-energy plasma electrons to the chamber walls, establishing a radial electric field. After the beam electrons emitted from the cathode traverse the chamber, they reach the end flange which can be either a beam dump, biased electrode, or a viewport. In addition to the existing 40 cm length ExB plasma reactor, a longer (100 cm) ExB Penning setup is currently under development at the PCRF.
A wide range of e-beam energy and flux regimes can be implemented in a range of working pressure regimes by implementing several different electron emitting cathodes. An ion-induced secondary electron emitting (iSEE) cathode can inject several mA of keV electrons into the discharge, sustaining a plasma down to the 10 mTorr pressure regime. We use a simple iSEE cathode design developed by the Naval Research Laboratory (NRL)1. For larger e-beam currents and lower pressures, we use PPPL-developed thermionic and RF cathodes which can generate e-beam currents of ~100mA-3000mA with electron energies of 10~100eV electrons.2,3
The e-beam generated ExB plasma setups can be used for fundamental studies of ExB plasma flow, characteristic instabilities and turbulence, plasma structures and cross-field transport. With available electrostatic and optical diagnostics and accessibility for these diagnostics, these sources offer excellent opportunity for comprehensive experimental validation of theories and numerical simulations.4–7
The e-beam can induce dissociation of gaseous molecular species, producing chemically active radical species useful for material processing applications (e.g.., graphene hydrogenation3 Fig. 2, hydrogen passivation of diamond substrates8). The axial magnetic field limits electron flux and the radial electric field limits the ion flux to the substrate, mitigating a potential ion-induced damage to substrate material.
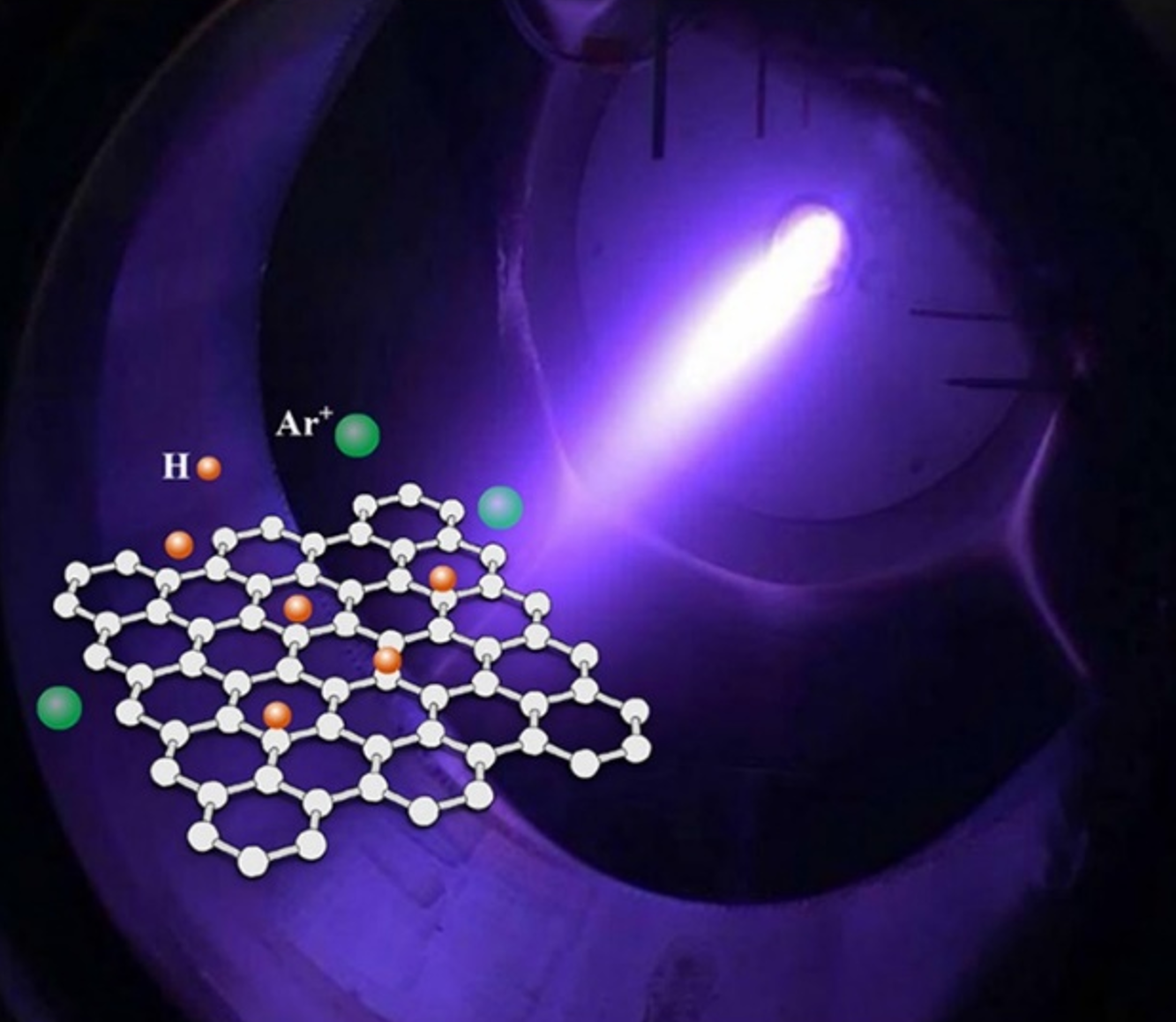
Figure 2. Hydrogenation of graphene by an e-beam plasma. H radicals are produced by an e-beam extracted from an RF cathode injected into a mix of Ar/H2.3
References
- S.G. Walton, D.R. Boris, S.C. Hernández, E.H. Lock, Tz.B. Petrova, G.M. Petrov, and R.F. Fernsler, Electron Beam Generated Plasmas for Ultra Low Te Processing, ECS J. Solid State Sci. Technol. 4, N5033 (2015).
- E. Rodríguez, V. Skoutnev, Y. Raitses, A. Powis, I. Kaganovich, and A. Smolyakov, Boundary-induced effect on the spoke-like activity in E x B plasma, Physics of Plasmas 26, 053503 (2019).
- F. Zhao, Y. Raitses, X. Yang, A. Tan, and C.G. Tully, High hydrogen coverage on graphene via low temperature plasma with applied magnetic field, Carbon 177, 244 (2021).
- J. Carlsson, I. Kaganovich, A. Powis, Y. Raitses, I. Romadanov, and A. Smolyakov, Particle-in-cell simulations of anomalous transport in a Penning discharge, Physics of Plasmas 25, 061201 (2018).
- A.T. Powis, J.A. Carlsson, I.D. Kaganovich, Y. Raitses, and A. Smolyakov, Scaling of spoke rotation frequency within a Penning discharge, Physics of Plasmas 25, (2018).
- O. Koshkarov, A. Smolyakov, Y. Raitses, and I. Kaganovich, Self-Organization, Structures, and Anomalous Transport in Turbulent Partially Magnetized Plasmas with Crossed Electric and Magnetic Fields, Physical Review Letters 6 (2019).
- Andrei Smolyakov (University of Saskatchewan, Canada) and Jean-Pierre Boeuf (LAPLACE-CNRS, France), PCRF User Project “The ExB Plasma Reference Cell for physics studies and codes validation”, runtime allocation in FY22.
- K-M. Fu (University of Washington), PCRF User Project “Cold hydrogen plasma passivation for quantum defect charge state control”, runtime allocation in FY21/22.
Hall thrusters and gridded ion sources
PCRF offers access to a set of laboratory plasma sources including 1-10 cm diameter Hall thrusters in the power range of 100-1000 W, and 3 cm gridded ion sources, as well as propulsion test facilities. For more details, please follow this link: http://htx.pppl.gov
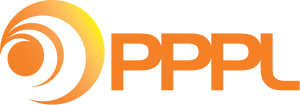